These 4 element are as follows:
1. Torque reaction from engine or propeller
2. Corkscrew effect
3. Gyroscopic action of the propeller
4. Asymmetrical loading of the propeller (P-factor)
This post will only discuss the first element, and the other three will follow in three separate posts.
TORQUE REACTION
This reaction involves Newton's Third Law of Physics - which states that for every action there is an equal and opposite reaction. Which translated to planes means that as the internal engine and propeller are rotating in one direction, an equal force is trying to rotate the aircraft in the opposite direction.
As you can see from the picture below, the propeller is turning in one direction labeled as "action", while the plane wings are rotating in the opposite direction labeled "reaction." Also note that the wings are trying to lift up on one side and down on the other.
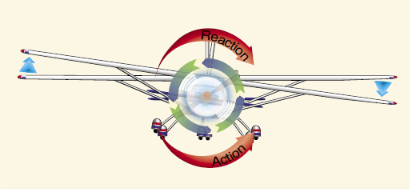
When the airplane is air born, this force is acting on the longitudinal axis, tending to make the airplane roll. Today's planes are designed with an engine offset to counteract this effect of torque.
When the plane is on the ground during takeoff roll, an additional turning tendency is induced by this torque reaction. As the left side of the plane is forced down, it is putting more weight on the left main landing gear. This effect results in more ground friction or drag, more on the left than the right causing even more turning to the left.
The extent of this increase depends on a few variables:
1. Size and horsepower of engine
2. Size of propeller and RPM
3. Size of the aircraft
4. Condition of the ground surface.
Next blog will cover Corkscrew Effect. Please tune in for more.